Resume
DNA-encoded library (DEL) screening revolutionizes early drug discovery through four decisive advantages. First, chemical scope: combinatorial synthesis paired with DNA barcoding empowers libraries of 108–1012 distinct small molecules, dwarfing traditional HTS collections. Second, efficiency: pooled selections require picomole amounts of compound and target, slashing reagent costs and cycle time. Third, data richness: next-generation sequencing yields highly quantitative enrichment profiles, enabling immediate structure–activity insights from a single binding experiment. Finally, versatility: DEL selections run against purified proteins, surface receptors, RNA, or in live cells, and can be rapidly iterated with off-DNA resynthesis and follow-up chemistry, accelerating translation from hit to lead.
Understanding DEL Screening: From Target Binding to Hit Validation
DNA-Encoded Library (DEL) technology has rapidly become a cornerstone in modern drug discovery. It enables researchers to screen millions of small molecules in a single tube, identify potent binders to a protein target, and validate those hits in record time—all while consuming minimal material and resources.
In this article, we provide a comprehensive walkthrough of the DEL screening process—from initial target engagement to hit confirmation. We’ll cover both classical techniques and novel advancements, including methods demonstrated in cutting-edge studies such as yoctoReactor (yR)-based homogeneous screening and in vivo DEL screening using Xenopus laevis oocytes.
What Is DEL Screening?
DNA-Encoded Libraries are a fusion of chemistry and molecular biology. Each small molecule in the library is covalently linked to a unique DNA tag that encodes the molecule’s synthetic pathway. This barcoding allows for high-throughput identification of binding molecules via next-generation sequencing. Vipergen utilizes its yoctoReactor (yR) technology to synthesize DELs with 100 % correspondence between DNA-barcode and the displayed small molecule.
DEL screening is fundamentally a “fishing expedition”. Instead of testing one molecule at a time, DEL allows millions of compounds to interact with a target simultaneously. After incubation, those compounds that bind to the protein of interest are enriched, recovered, and identified through DNA sequencing.
The result? A faster, more efficient, and more scalable approach to early hit discovery than traditional high-throughput screening (HTS).
Step-by-Step Overview of DEL Screening
- Target Identification: Clarify the biological question, then select a purified protein, protein complex, or cell-surface presentation that is drug-relevant, structurally characterized, and obtainable at ≥95 % purity. Confirm catalytic activity or ligand binding by an orthogonal assay (e.g., SPR or enzymatic turnover). Map allosteric and orthosteric sites, note essential cofactors, and define screening conditions (pH, salt, detergent, divalent ions) that keep the target stable ≥24 h at 4 °C. Reserve at least 0.5–1 mg for selections. Alternatively, skip most of these steps and utilize Vipergen’s Cellular Binder Trap Enrichment here.
- Library Synthesis: A wide range of chemical reactions have over the past two years been adapted to be compatible with DEL synthesis (Read about DNA compatible chemical reactions here). DELs are usually synthesized by split-and-pool combinatorial chemistry, but other methods such as Vipergen’s Yoctoreactor also exist.
- Selection & Amplification: Incubate the encoded library with immobilized or soluble target under near-physiological buffer for 1–2 h. Separate binders via affinity capture or size-exclusion, then wash stringently to remove nonspecific DNA. Elute bound complexes (heat, imidazole, competitive ligand). PCR-amplify recovered DNA, re-associate with its small-molecule (if required), and repeat 2–4 selection rounds, gradually increasing wash stringency.
- Hit Decoding: Deep-sequence the final PCR pool (Illumina or similar). Translate DNA barcodes to chemical structures with the synthesis map. Quantify enrichment by comparing read counts to the naïve library; typical meaningful enrichments are >10- to 1000-fold. Use clustering to identify chemotypes and remove PCR or sequencing artefacts. Output a ranked hit list with calculated phys-chem properties and any known PAINS alerts flagged.
- Biological and Chemical Validation: Resynthesize top 20–200 compounds off-DNA at ≥95% purity. Confirm identity by LC-MS and NMR. Test binding/functional activity in orthogonal biophysical (SPR, ITC, DSF) and cellular assays, determining KD/IC50. Evaluate selectivity across close homologues and ADME liabilities (microsomal stability, CYP inhibition). Prioritize hits showing reproducible potency, clean spectra, and tractable chemistry for follow-up medicinal-chemistry optimization.
Step 1: Target Binding – Classical In Vitro Methods
Immobilization-Based DEL Screening
Figure 1: Affinity bases DEL Screening by incubation of DEL with a POI immobilized on beads. Following elution the DNA is PCR amplified and sequenced.
Pros:
- Easy to implement in most labs
- Useful for well-characterized, stable proteins
Challenges:
- Immobilization can distort the protein’s native conformation
- The washing steps may strip off weak but potentially valuable binders
- Nonspecific binding to the matrix can generate false positives
This method laid the groundwork for more refined techniques and remains useful in certain contexts. Although new methods exist, variations to this protocol (e.g. immobilizing the POI after incubation with DEL) is still the method of choice for many companies.
Homogeneous Assays – A Leap Forward in DEL Screening
To overcome the pitfalls of immobilization, homogeneous DEL screening methods were developed. One standout is Vipergens Binder Trap Enrichment (BTE), a technology that enables protein and DEL to interact freely in solution without immobilization (Petersen 2016).
Here’s how it works:
- The target protein is tagged with DNA and incubated with the DEL in solution.
- This mixture is diluted and emulsified into millions of microscopic water-in-oil droplets.
- Each droplet acts as an individual test tube. Statistically, only those containing a true binding complex (protein + DEL molecule) will consistently appear.
- Within each droplet, a ligation reaction joins the protein DNA and DEL DNA, thereby encoding the interaction.
- After breaking the emulsion, only ligated DNA products (i.e., true binders) are PCR-amplified and sequenced.
Case story – p38α kinase inhibitors identified from in vitro DEL Screening
Early work from Vipergen explored the utilization of Binder Trap Enrichment (BTE), which circumvents the need to immobilize the target protein of interest. In brief equilibrium is established between the DEL and a DNA tagged protein, which undergoes a dissociation phase triggered by a high factor dilution and formation of emulsions by addition of oil. Droplets containing both DNA-tagged POI and a library member can be ligated, PCR amplified, and sequenced by NGS to identify novel binders.
Utilizing this DEL screening workflow researchers managed to identify potent nanomolar inhibitors of the p38α MAP kinase. Besides inhibition assays of the hit compounds, hit validation was done by both performing selectivity assays against a panel of 99 kinases as well as obtaining crystal structures of inhibitors bound to p38α (Petersen 2016).
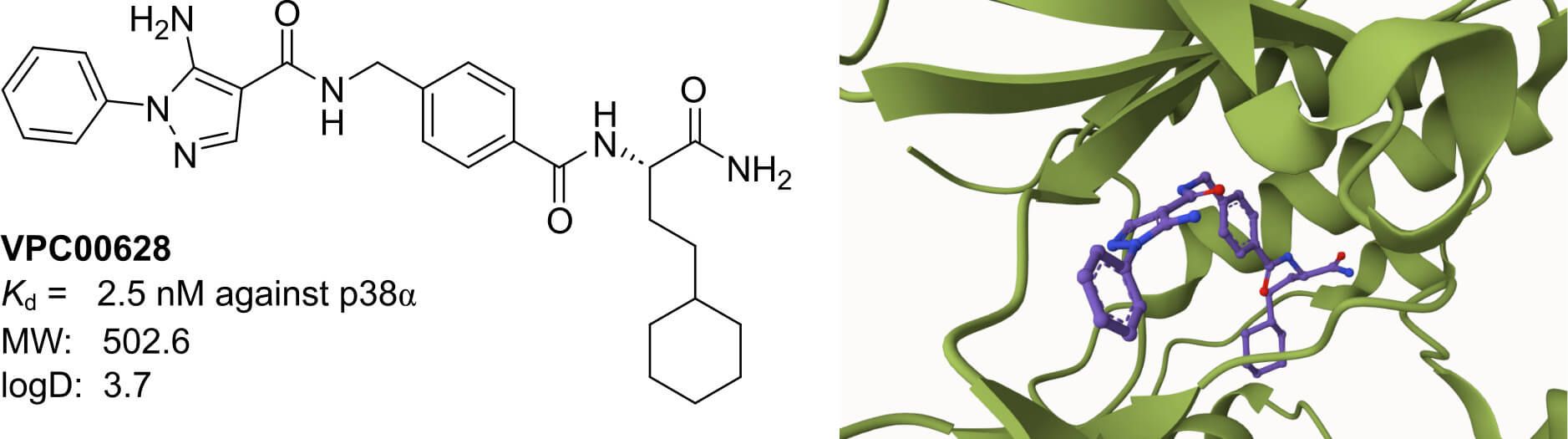
Figure 2: Structure of VPC00628 and its binding to p38α (PDB: 5LAR).
A 2016 study using this method identified a highly potent p38α kinase inhibitor (IC₅₀ = 7 nM) from a 12.6-million-member DEL in a single round of screening (Petersen 2016).
Besides Vipergens BTE-technology, Li and colleagues developed a method for DNA crosslinking in which a single-stranded DEL is incubated with the POI in solution. Addition of a complementary DNA strand with a photocrosslinking handle allows for selective amplification of binding DEL-members (Zhao 2014). Other methods exist including the use of capillary electrophoresis which allows for the isolation of target-binder complexes (Huang 2022).
Step 2: Hit Identification and Data Analysis
Once the screening is done, the DNA tags from the retained compounds are sequenced and decoded. This generates a list of potential hits—molecules that bound to the protein.
To make sense of this data:
- Signal plots are used to visualize how often each compound is observed. True binders show up repeatedly, while random hits appear infrequently.
- Statistical thresholds (like log-linear cutoffs) are applied to separate “signal” from “noise”.
- Chemoinformatics tools such as Tanimoto similarity scoring are used to cluster hits into chemical families. This helps identify recurring structural motifs that might drive binding to give access to structure activity relationship (SAR).
This analytical layer is critical. It transforms raw sequencing reads into actionable drug discovery leads.
In the yoctoReactor p38α screening study, for example, 236 primary hits were identified, clustered into several structure families, and a subset of 24 compounds was resynthesized off-DNA for further testing—22 of which were confirmed active (Petersen 2016).
Step 3: Hit Validation – A Multi-Pronged Approach
Once hits are identified through DEL screening, the next—and arguably most critical—phase is hit validation. This step ensures that the DNA-identified compounds truly bind the target of interest, act through the desired mechanism, and have the potential to be optimized into viable drug candidates.
Initially, selected hit compounds are synthesized without the DNA-barcode, to ensure that the expected signals arise from the small molecule. Either during the screening process or immediately after, counter screens against expected off-target proteins are run. Hit validation typically involves a tiered approach, beginning with biochemical confirmation, followed by biophysical binding assays, cellular engagement studies, and structural characterization.
Here’s a breakdown of the key techniques:
1. Biochemical Assays: Functional Confirmation
These assays confirm whether a compound modulates the activity of the target protein in vitro.
- Enzyme inhibition assays (e.g., ADP-Glo™, radiometric assays): Quantify the ability of a hit to inhibit enzymatic activity, providing IC₅₀ values.
- Fluorescence polarization (FP) or TR-FRET: Measure binding or displacement of a fluorescent probe in a functional context.
- AlphaScreen/AlphaLISA: Measurement of molecular interactions in proximity-based formats, often used for protein-protein interaction targets.
Screening and/or validation against native protein along with inactive protein mutants can help to demonstrate that the activity is due to specific, rather than nonspecific, binding.
2. Biophysical Assays: Direct Binding Validation
These techniques measure the physical interaction between the hit and its target—independently of functional readouts.
- Surface Plasmon Resonance (SPR): Gold standard for real-time binding kinetics (on-rate, off-rate, KD). Ideal for confirming target specificity and binding strength.
- BLI (Bio-layer interferometry): Can also provide real-time binding kinetics, but is often most used for larger compounds.
- Isothermal Titration Calorimetry (ITC): Measures heat released/absorbed during binding, providing affinity and thermodynamics (enthalpy, entropy).
- Differential Scanning Fluorimetry (DSF/Thermal Shift Assay): Detects ligand-induced stabilization of protein structure via shits in melting point.
- Microscale Thermophoresis (MST): Measures molecule movement in a temperature gradient to infer binding—requires minimal sample and is suitable for challenging targets.
3. Target Engagement in Cells: Is It Binding in the Right Place?
Validating that a compound binds the target inside cells is critical for assessing cell permeability, target engagement, and selectivity.
- Cellular Thermal Shift Assay (CETSA): Detects stabilization of proteins upon ligand binding inside cells or lysates. A powerful method for confirming intracellular engagement without needing modifications. Albeit looking similar to DSF, CETSA gives information about cellular uptake of the compound and its capability of engaging with the target in a cellular context.
- FRET/BRET (Förster/Bioluminescence Resonance Energy Transfer): Monitors binding-induced changes in proximity between fluorophores attached to the compound or protein. Useful for both in vitro and live-cell readouts.
- NanoBRET™: An advanced live-cell assay platform using NanoLuc-tagged targets and fluorescent tracers to measure direct engagement in real time.
Target degradation (e.g., PROTAC activity): Functional confirmation that binding results in downstream effects, such as ubiquitination and proteasomal degradation.
4. Structural Characterization: Seeing Is Believing
Structural studies offer atomic-level insight into the binding mode of a compound. These methods are invaluable for lead optimization and SAR development.
- X-ray Crystallography: Provides high-resolution structures of protein-ligand complexes. Particularly useful for kinase targets and other crystallizable proteins.
- Cryo-Electron Microscopy (Cryo-EM): Revolutionizing structure-based drug discovery for larger or difficult-to-crystallize targets, such as membrane proteins or protein complexes.
- NMR Spectroscopy: Suitable for studying smaller proteins or flexible binding interactions, especially for fragment-based hits.
Mass Spectrometry (HDX-MS or Native MS): Useful for mapping binding epitopes and conformational changes upon compound interaction.
5. In Vivo Models (Advanced Stage)
Though not always part of early validation, in vivo pharmacology helps confirm whether the hit is bioavailable, efficacious, and safe:
- Mouse xenograft models for oncology targets
- Zebrafish screening for CNS or cardiovascular hits
- PK/PD profiling in rodents or non-human primates
The Power of a Layered Approach
Each of these methods provides a different lens through which to examine a hit compound. By combining them, researchers can build a robust profile of hit quality—not just potency, but selectivity, binding mode, mechanism of action, and cellular relevance.
The stronger and more multi-dimensional this profile, the higher the confidence in taking a compound forward into lead optimization and preclinical development.
Emerging Strategies in DEL Screening
Many cellular targets including membrane proteins are challenging to screen utilizing classical in vitro selection methods. Previous methods have involved isolating and screening the soluble domains or screening against full length membrane proteins trapped in lipid nanodisks (Huang 2022). More recently, approaches have been developed, to screen transmembrane targets in the extracellular domain of live cells. To reach a high enough concentration of target, overexpression might be necessary. Alternative approaches include the installation of a DNA tag on the target, which can guide library hybridization and promote ligand binding (Huang 2021).
Albeit appealing, the above-mentioned methods only allow for the screening of membrane targets on the extra cellular part of the cell, which limits the methods.
DEL Screening Inside Living Cells
Screening of DELs inside living cells is highly attractive as it allows for the screening against proteins in their native environment, which allows conservation of biological features including post-translational modifications. The main challenge associated with screening DELs inside living cells is library delivery, as the DNA-tag is not cell permeable. The first attempt of screening inside cells was performed by Cai and co-workers, who constructed a DNA Encoded Library where each individual member was conjugated to a cyclic, cell-penetrating peptide (cCPP). Their results indicated that limiting the DNA to 60 base pairs allowed for around 11% cell entry. Longer DNA chains of 140 base pairs significantly reduced the cellular uptake to around 1%. 20-200 fold enrichment of peptide controls was generally observed when they tested against a non-cCPP labeled library members (Cai 2019).
Case study – DEL Screening inside living cells
In 2021, Vipergen broke new ground when in vivo DEL screening inside living cells was introduced (Petersen 2021). Using Xenopus laevis oocytes (frog eggs), researchers microinjected:
- mRNA encoding the target protein fused to a “prey” tag
- DNA encoded library
- A bait-DNA strand with affinity to the prey
Inside the cell, DEL molecules bind to their target in a fully native cellular environment. After incubation, the cell is lysed, and BTE is performed on the contents. This method—cellular Binder Trap Enrichment (cBTE)—preserves physiological relevance and eliminates the need for purified proteins. Furthermore, it allows for the screening of all proteins which can be expressed in cells, including receptors and other proteins found in the cell membrane.
In a single study, 194 million-member DELs yielded validated hits for p38α, ACSS2, and DOCK5—proving that DEL screening can now target proteins that were previously “undruggable” due to expression or stability challenges.
Why DEL Screening Should Be Part of Your Drug Discovery Strategy
-
High throughput: Screen up to billions of compounds simultaneously
-
Low resource use: Minimal protein and reagent consumption
-
Rapid turnaround: Go from idea to validated hits in weeks
-
Cost-effective: No need to build in-house screening infrastructure
-
Novelty-driven: Discover unique binding chemotypes not found in legacy libraries
By partnering with Vipergen that specializes in DEL synthesis and screening, you can immediately access this high-powered discovery engine.
DEL Screening as a Catalyst for Sustainable Drug Discovery and Development
Sustainable drug discovery seeks to generate therapeutic breakthroughs while minimizing ecological footprints, conserving resources, and improving social outcomes across the R&D pipeline (Vidaurre 2024). By embedding life cycle thinking – energy, water, waste, and animal use – into every experimental choice, organizations can align innovation with global sustainability goals and regulatory expectations (Castiello 2023).
DEL Screening technologies embodies this ethos from the initial experiments. A single tube or cell can screen hundreds of millions of compounds at femtomole scale, displacing the multi-plate, multi-liter workflows of traditional HTS. The result is dramatic reductions in solvent consumption, plastic waste, and purified protein – key metrics for sustainable drug discovery programs.
DEL synthesis operates largely in aqueous buffers at ambient temperature, adheres to catalytic reagent loading, and avoids toxic halogenated solvents. These practices mirror multiple of the Twelve Principles of Green Chemistry (Anastas & Warner 1998, Anastas & Eghbali 2009), directly supporting sustainable drug development. Furthermore, the DNA barcode serves as a synthetic record: once a high-affinity hit is decoded, milligram quantities can be synthesized readily without route scouting or large-scale pilot batches, conserving reagents and logistics.
Because DEL screening campaigns deliver rich structure activity relationship data earlier, drug discovery teams can “fail fast” in vitro, cutting down exploratory animal studies and energy-intensive scale-ups (Lendrem 2013, Gorzalczany 2020). Taken together, DEL screening converts sustainability from an aspirational slogan into quantifiable advantage – proving that high-impact science and sustainable drug development can progress hand in hand.
12 Principles of Green Chemistry
-
Prevent Waste
-
Atom Economy
-
Less Hazardous Synthesis
-
Design Benign Chemicals
-
Benign Solvents & Auxiliaries
-
Design for Energy Efficiency
-
Use of Renewable Feedstocks
-
Reduce Derivatives
-
Catalysis
-
Design for Degradation
-
Real-Time Analysis for Pollution Prevention
-
Inherently Benign Chemistry for Accident Prevention
Conclusion
From classic solid-phase techniques to cutting-edge cellular assays, DEL screening is reshaping the early drug discovery landscape. Technologies like BTE and cBTE are not just incremental upgrades, they’re paradigm shifts.
If you’re looking to kickstart a drug discovery campaign, identify novel chemical matter, or target previously intractable proteins, DEL screening is one of the smartest starting points available today.
Ready to explore DEL screening for your target of interest?
Let’s talk about how our diversity-oriented high-fidelity libraries and our screening workflows can give you an early lead!
FAQ
High fidelity (i.e. 100 % correspondence between codon DNA and the BB encoded) is important to remove potential truncates from the DEL, which can cause to a lot of noise in the data and eventually lead to false positives. Read how Vipergen synthesize their DELs without any truncates here
DEL screening in cells can be used for all drug targets but is especially useful when targeting proteins which are difficult to express or purify. This could be when targeting protein complexes, integral membrane proteins and transcription factors. Furthermore, Vipergen’s DEL Screening in cells allows for the direct discovery of molecular glues (DELs in Cells – Molecular Glue Direct), PPI inhibitors (DELs in Cells – PPI inhibitor Direct) and access to selectivity data directly (DELs in Cells – Selective Direct).
References
- Cai, B. et. al. 2019, Selection of DNA-Encoded Libraries to Protein Targets within and on Living Cells, J. Am. Chem. Soc., 141, 17057-17061. doi.org/10.1021/jacs.9b08085
- Gironda-Martínex, A. et. al., 2021, DNA-Encoded Chemical Libraries: A Comprehensive Review with Succesful Stories and Future Challenges, ACS Pharmacol. Transl. Sci., 4, 1265-1279. doi.org/10.1021/acsptsci.1c00118
- Huang, Y. et. al., 2021, Selection of DNA-encoded chemical libraries against endogenous membrane proteins on live cells, Nat. Chem., 13, 77-88. doi.org/10.1038/s41557-020-00605-x
- Huang, Y. et. al., 2022, Strategies for developing DNA-encoded libraries beyond binding assays, Nat. Chem., 14, 129-140. doi.org/10.1038/s41557-021-00877-x
- Petersen, L. K. et. al., 2016, Novel p38α MAP kinase inhibitors identified from yoctoReactor DNA-encoded small molecule library, Med. Chem. Commun., 7, 1332-1339. doi.org/10.1039/c6md00241b
- Petersen, K. K. et. Al., 2021, Screening of DNA-Encoded Small Molecule Libraries inside a Living Cell, J. Am. Chem. Soc., 143, 7, 2751-2756. doi.org/10.1021/jacs.0c09213
- Zhao, P. et. al., 2014, Selection of DNA-encoded small molecule libraries against unmodified and non-immobilized protein targets. Angew. Chem. Int. Ed., 53, 38, 10056-10059. doi.org/10.1002/anie.201404830
- Anastas, P. T. and Eghbali, N., Green Chemistry: Principles and Practice, Chem. Soc. Rev., 2010, 39, 301-312. doi.org/10.1039/B918763B
- Anastas, P. T. and Warner, J. C., Green Chemistry: Theory and Practice, Oxford University Press, New York, 1998. doi.org/10.1093/oso/9780198506980.001.0001
- Castiello, C. et. al., GreenMedChem: the challenge in the next decade toward eco-friendly compounds and processes in drug design, Green Chem., 2023, 25, 2109-2169. doi.org/10.1039/d2gc03772f
- Gorzalczany, S. B. and Basso, A. G. R., Strategies to apply 3Rs in preclinical testing, Pharmacol. Res. Perspect., 2021, 9, e00863. doi.org/10.1002/prp2.863
- Lendrem, D. W. and Lendrem, B. C., Torching the Haystack: modelling fast-fail strategies in drug development, Drug Discov. Today, 2013, 18, 331-336. doi.org/10.1016/j.drudis.2012.11.011
- Vidaurre, R. et. al., Design of greener drugs: align parameters in pharmaceutical R&D and drivers for environmental impact, Drug Discov. Today, 2024, 29, 104022. doi.org/10.1016/j.drudis.2024.104022
Related Services
Service | |
---|---|
Small molecule drug discovery for even hard-to-drug targets – identify inhibitors, binders and modulators | |
Molecular Glue Direct | |
PPI Inhibitor Direct | |
Integral membrane proteins | |
Specificity Direct – multiplexed screening of target and anti-targets | |
Express – optimized for fast turn – around-time | |
Snap – easy, fast, and affordable |